There is an ever-increasing demand for accurate, yet low-cost and highly reliable guidance, control, and navigation systems for air, land, sea, and space vehicles. The heart of these systems are gyroscopes, devices which can precisely measure changes in orientation of an airplane, ship, tank, or satellite as it moves. The familiar mechanical gyroscope with its rotating wheels is now seeing competition from the laser gyroscope, another application of the versatile laser. For this reason, military readers may find it helpful to know how the laser gyroscope works, its advantages and disadvantages, the current status of laser gyroscope technology, and what it all means in terms of future military system capability.
How a Laser Gyroscope Works
The laser gyroscope works on a physical principle discovered by the French physicist G. Sagnac in the first decade of this century. In simple terms, Sagnac found that the difference in time that two beams, each traveling in opposite directions, take to travel around a closed path mounted on a rotating platform is directly proportional to the speed at which the platform is rotating. This principle is incorporated in a laser gyroscope. Although Sagnac and other scientists demonstrated the concept in the laboratory, it was not until the 1960s, with the advent of the laser beam with its unique properties, that the principle could be used in a practical gyroscope. The key properties of the laser that make the laser gyroscope possible are the laser's coherent light beam, its single frequency, its small amount of diffusion, and its ability to be easily focused, split, and deflected.
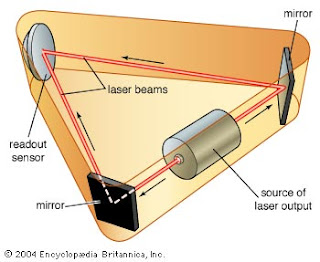
Mirrors are located at each corner to turn the beams. At one corner, there is a detector or an output sensor. However, rather than detecting time-of-travel differences, the detectors measure differences in frequency, using the Doppler principle which is the basis of range-finding radars. The beam that is traveling in the direction of rotation of the platform has a longer distance to travel and thus a lower frequency. Conversely, the beam traveling against the direction of motion has a shorter path and a higher frequency. The difference in frequency is directly proportional to the rotation rate.
In an actual application Such as an aircraft autopilot, three laser gyroscopes would be used to sense changes in pitch, roll, and yaw. In addition, there would be three accelerometers to measure longitudinal, lateral, and vertical motion.
Advantages and Disadvantages
There are many characteristics desired in a gyroscope for military applications. These include accuracy, long-term stability, low cost, high reliability, low maintenance, high tolerance to accelerations and vibration, small size and light weight, minimum start-lip time, and low power requirements.
One of the significant attributes of the laser gyro is its use of very few moving parts. Indeed, it is theoretically possible to build laser gyros without any moving components. Unlike the conventional spinning gyroscope with its gimbals, bearings, and torque motors, the laser gyroscope uses a ring of laser light, together with rigid mirrors and electronic devices. Thus the laser gyroscope is more rugged than conventional gyros, offering the obvious advantages of much greater reliability and lower maintenance requirements. Typically, laser gyros have a mean-time between failures about twice that found in conventional gyros [1]. Not only does the greater reliability of the laser gyro mean lower life-cycle costs, but such gyros potentially could be less costly to produce in the first place. Current technological efforts are under way to get production costs down. Indeed, some of the advanced work on very small solid-state devices portends substantial reduction in cost and increases in reliability. The miniature laser gyros that may result could be used in such applications as low-cost tactical missiles and even "guidance" systems issued to the individual foot soldier to replace his compass.
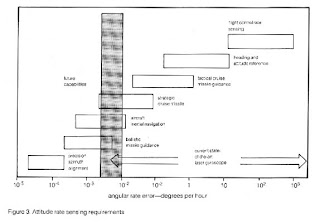
In regard to the important matter of accuracy, the laser gyro has the potential to provide accuracy equivalent to that offered by mechanical gyroscopes, even to the accuracy levels required for the ballistic missile role [3].
Today, accuracy levels of laser gyros in production are in the range of slightly less than one nautical mile per flight hour––about the minimum required for typical aircraft missions and for use in tactical cruise missiles. Short-range tactical missiles such as the AIM-7 and AIM-9 can do very well with rate gyros in the 10-nm/hr to 100-nm/hr class.
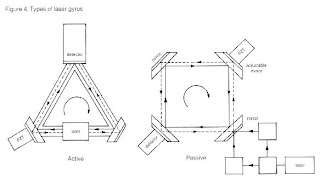
Applications and the Future of the Laser Gyroscope
Laser gyroscopes are more than a laboratory experiment. A laser gyroscope system built by Honeywell is used on the Boeing 757 and 767, the new generation of commercial transports. The European A310 Airbus uses a laser gyro unit built by Litton. Honeywell's laser gyro navigation systems are now being installed in business jets such as the Gulfstream. Other prototype laser gyros have been test flown on commercial aircraft, military fighters such as the A-7E and F-14, and helicopters, giving good accuracy and outstanding reliability.
The future for the laser gyroscope is a bright one. A recent marketing survey has shown that in the last half of this decade about 50 percent of the dollars spent on gyros for military aircraft will go for the laser variety. In the 1990s, the amount will jump to 75 percent. According to this study, laser gyros should start appearing in tactical missiles during the late 1980s or early 1990s. By the mid-1990s, they will capture a predominant share of the market. The laser gyroscope is a viable contender for almost all military and commercial applications, including military and commercial aircraft, tactical and strategic missiles, naval and marine vehicles, land vehicles and weapon platforms, and spacecraft [4].
References
[1] John C. Patterson, "Laser Gyros, Supplanting Inertial Navs," Defense Electronics, May 1981, pp. 106-09.
[1] John C. Patterson, "Laser Gyros, Supplanting Inertial Navs," Defense Electronics, May 1981, pp. 106-09.
[2] Jerry Lockenour and John J. Deyst, Jr., "Aerospace Highlight 1980, Guidance and Control," Astronautics and Aeronautics, December 1980, pp. 58-59.
[3] W. Kent Stowell, Robert W. McAdory, and Robert Ziernicki," Air Force Applications for Optical Rotation Rate Sensors," Proceedings of the SPIE Laser Inertial Rotation Sensors, vol. 157, 30-31 August 1978, pp. 66-71.
[4] Robert G. Brown, "Growing Role Predicted for Inertial Technology," Military Electronics/Countermeasures, December 1980, pp. 40-46.
No comments:
Post a Comment